Designing Resilient Infrastructure for Earthquake-Prone Regions: Building for Safety and Sustainability
Earthquakes are among the most destructive natural disasters, posing significant risks to human life, property, and economies. For regions prone to seismic activity, designing resilient infrastructure is critical to minimizing damage, ensuring public safety, and fostering long-term economic stability. Resilient infrastructure not only withstands seismic events but also enables faster recovery and reduces the need for costly repairs.
This article explores the principles, innovations, and challenges of designing earthquake-resilient infrastructure, emphasizing the importance of preparedness and sustainability in seismic regions.
Understanding the Impact of Earthquakes on Infrastructure
Earthquakes generate powerful ground motions that can cause buildings, bridges, roads, and utilities to collapse or become severely damaged. Key factors contributing to infrastructure vulnerability include:
- Ground Shaking: The intensity and duration of ground shaking can weaken structures, leading to failures in foundations, walls, and supports.
- Soil Liquefaction: In certain soil conditions, intense shaking can cause the ground to behave like a liquid, undermining the stability of structures.
- Surface Ruptures: The displacement along fault lines can damage roads, pipelines, and buildings.
- Aftershocks: Secondary tremors often exacerbate damage and hinder recovery efforts.
Principles of Earthquake-Resilient Design
Designing infrastructure for earthquake-prone regions involves adhering to specific principles aimed at enhancing structural performance during seismic events. These include:
1. Flexibility and Ductility
Structures must be designed to absorb and dissipate seismic energy without collapsing. Materials such as reinforced concrete and structural steel provide flexibility and ductility, allowing buildings to deform without catastrophic failure.
2. Seismic Isolation
Seismic isolation systems, such as base isolators, decouple a structure from ground motion. These systems use flexible bearings or sliders to reduce the transfer of seismic forces, protecting the building’s integrity.
3. Reinforced Foundations
Strong and well-reinforced foundations are essential for maintaining stability during an earthquake. Deep foundations, such as pile systems, provide additional support in areas prone to soil liquefaction.
4. Symmetry and Uniformity
Structures with symmetric and uniform layouts perform better during earthquakes. Irregular shapes or uneven weight distribution can create weak points that amplify damage.
5. Redundancy in Design
Incorporating redundancy ensures that if one component fails, others can carry the load, preventing a total collapse.
6. Lightweight Materials
Using lightweight construction materials reduces the seismic load on structures, minimizing the risk of collapse.
Innovative Technologies in Earthquake-Resilient Infrastructure
Advances in engineering and technology have led to the development of innovative solutions for enhancing seismic resilience.
1. Smart Materials
Smart materials, such as shape-memory alloys and self-healing concrete, adapt to changing conditions and recover from damage, enhancing the durability of structures.
2. Energy Dissipation Devices
Dampers and shock absorbers dissipate seismic energy, reducing the forces transmitted to the structure. Examples include tuned mass dampers and viscous fluid dampers.
3. Seismic-Resistant Building Codes
Modern building codes integrate the latest research and engineering practices to ensure structures are designed to withstand seismic forces. Regular updates to these codes reflect advancements in technology and understanding of seismic behavior.
4. Earthquake Early Warning Systems
Integrating early warning systems into infrastructure design allows for automated responses, such as shutting down gas lines, stopping trains, or activating emergency protocols before the seismic waves arrive.
5. Modular and Prefabricated Structures
Prefabricated components with built-in seismic features offer faster construction and enhanced performance during earthquakes.
Case Studies: Resilient Infrastructure in Action
1. Tokyo Skytree, Japan
The Tokyo Skytree, one of the tallest towers in the world, incorporates a central reinforced column and seismic dampers to withstand earthquakes and typhoons. The design is inspired by traditional Japanese pagodas, known for their earthquake resilience.
2. Bay Bridge, California
The San Francisco-Oakland Bay Bridge features seismic isolation bearings and energy-dissipating devices that allow it to flex and absorb ground motion during earthquakes.
3. Christchurch Rebuild, New Zealand
Following the devastating 2011 earthquake, Christchurch implemented innovative seismic design techniques, including base isolation and reinforced foundations, to rebuild safer and more resilient structures.
Challenges in Earthquake-Resilient Infrastructure Design
While significant progress has been made, several challenges remain in implementing earthquake-resilient designs:
- High Costs: Advanced materials, technologies, and engineering practices can be expensive, limiting their accessibility in developing regions.
- Aging Infrastructure: Retrofitting older buildings and bridges to meet modern seismic standards is often complex and costly.
- Lack of Expertise: In some regions, a shortage of skilled engineers and architects with expertise in seismic design poses a barrier to resilience efforts.
- Urban Density: Densely populated cities often lack the space for large-scale retrofitting or reconstruction projects.
Future Directions in Earthquake-Resilient Design
The future of earthquake-resilient infrastructure lies in leveraging technology, fostering collaboration, and adopting a proactive approach to seismic risk management. Key trends include:
- AI and Machine Learning: Advanced algorithms can analyze seismic data, predict structural performance, and optimize designs for resilience.
- Sustainability Integration: Combining earthquake-resilient features with sustainable design practices ensures long-term environmental and economic benefits.
- Global Collaboration: Sharing knowledge, technologies, and resources across borders enhances the capacity to build safer communities worldwide.
- Community Involvement: Engaging local communities in planning and preparedness efforts fosters resilience and awareness at the grassroots level.
Conclusion: Building a Safer Future
Designing resilient infrastructure for earthquake-prone regions is both a scientific and humanitarian imperative. By integrating cutting-edge engineering, innovative technologies, and sustainable practices, we can reduce the devastating impacts of earthquakes and build communities that are safer and more prepared for the challenges of the future.
As we continue to advance our understanding of seismic behavior and develop new tools for resilience, the goal of creating earthquake-resistant infrastructure becomes increasingly achievable—offering hope for a safer, more sustainable world.
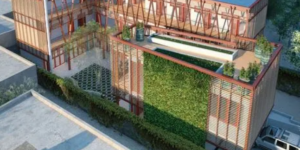
Also Read :
- The Importance of Geotechnical Engineering in Infrastructure Development
- Advances in Cybersecurity Engineering for IoT Devices
- The Role of Bioplastics in Sustainable Product Design
- High-Performance Computing in Chemical Engineering Simulations
- The Internet of Things (IoT) in Structural Health Monitoring